Why are red blood cells (RBCs) so good at transporting oxygen?
Once oxygen enters the pulmonary capillaries from the alveoli, a portion of the oxygen remains in the plasma while most of it enters the red blood cells (RBCs).
RBCs
The red blood cells are derived from bone marrow progenitor cells. With maturation, the red cell loses its nucleus, ribosomes, and mitochondria. This means the red cell can’t divide, conduct oxidative phosphorylation, or synthesize proteins.But it can function as a very effective transporter. The concave shape provides a large surface area; the membrane, a typical bilayer, is pliable and relatively durable allowing it to fold on itself and fit through the tiny capillaries. In addition, the red cell’s membrane facilitates oxygen entry into the cell. Lastly, and perhaps most importantly, the red blood cells also contain hemoglobin.
Become a great clinician with our video courses and workshops
Hemoglobin
Each molecule of hemoglobin contains a globin protein and heme, which is a complex of iron and porphyrin.The globin protein in a single hemoglobin molecule is a tetramer of polypeptides, including two alpha and two non-alpha types. The major hemoglobin in adults, Hemoglobin A1, consists of two alpha and two beta chains (alpha2 / beta2). The minor adult hemoglobin, Hemoglobin A2, consists of two alpha and two delta chains (alpha2 / delta2). Each of the subunit moieties binds non-covalently with the others.
The heme component of hemoglobin consists of a single ferrous ion (Fe2+) chelated to a porphyrin ring known as protoporphyrin IX. A single oxygen molecule binds to each heme moiety within each of the four protein chains. The oxygen molecules bind avidly, but reversibly, enabling each hemoglobin molecule to carry four molecules of oxygen.Hemoglobin loaded with a full complement of oxygen is known as oxyhemoglobin; hemoglobin without oxygen, or without a full complement of oxygen, is known as deoxygenated hemoglobin or deoxyhemoglobin.
When isolated from the heme proteins, ionic ferrous heme binds oxygen irreversibly, producing oxidized, ferric heme (Fe+++), a complex which prevents oxygen unloading in peripheral tissues. The special arrangement of heme and proteins within hemoglobin prevents ferric ion formation, facilitating reversible oxygen loading and unloading.That’s it for now. If you want to improve your understanding of key concepts in medicine, and improve your clinical skills, make sure to register for a free trial account, which will give you access to free videos and downloads. We’ll help you make the right decisions for yourself and your patients.
Recommended reading
- Grippi, MA. 1995. “Gas exchange in the lung”. In: Lippincott's Pathophysiology Series: Pulmonary Pathophysiology. 1st edition. Philadelphia: Lippincott Williams & Wilkins. (Grippi 1995, 137–149)
- Grippi, MA. 1995. “Clinical presentations: gas exchange and transport”. In: Lippincott's Pathophysiology Series: Pulmonary Pathophysiology. 1st edition. Philadelphia: Lippincott Williams & Wilkins. (Grippi 1995, 171–176)
- Grippi, MA and Tino, G. 2015. “Pulmonary function testing”. In: Fishman's Pulmonary Diseases and Disorders, edited by MA, Grippi (editor-in-chief), JA, Elias, JA, Fishman, RM, Kotloff, AI, Pack, RM, Senior (editors). 5th edition. New York: McGraw-Hill Education. (Grippi and Tino 2015, 502–536)
- Tino, G and Grippi, MA. 1995. “Gas transport to and from peripheral tissues”. In: Lippincott's Pathophysiology Series: Pulmonary Pathophysiology. 1st edition. Philadelphia: Lippincott Williams & Wilkins. (Tino and Grippi 1995, 151–170)
- Wagner, PD. 2015. The physiologic basis of pulmonary gas exchange: implications for clinical interpretation of arterial blood gases. Eur Respir J. 45: 227–243. PMID: 25323225
Become an expert
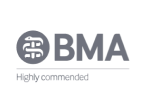
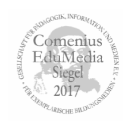