How do I interpret a Davenport diagram?
The relationship among the partial pressure of carbon dioxide in the arteries, bicarbonate concentration, and pH may be depicted graphically in a Davenport diagram.
In the Davenport diagram shown, plasma bicarbonate concentration is plotted against pH at a variety of levels of arterial carbon dioxide tension (isobars). Point A depicts the normal values for arterial pH, arterial carbon dioxide tension, and bicarbonate concentration. Point B represents the relationships when carbonic acid is added to blood (e.g., by increasing arterial carbon dioxide tension). Point C represents the relationships when carbonic acid is removed from blood (e.g., by decreasing arterial carbon dioxide tension). The line connecting Points A, B, and C defines the buffer line for arterial blood.
Changes in arterial carbon dioxide tension
Alkalemia, or an arterial pH > 7.44, arises when, in the setting of a constant plasma bicarbonate concentration, arterial carbon dioxide tension is reduced, as represented by a shift in arterial carbon dioxide tension from Point A to an arterial carbon dioxide tension isobar to the right of Point A.
Acidemia, or an arterial pH < 7.36, arises when, in the setting of a constant arterial bicarbonate concentration, arterial carbon dioxide tension is increased, reflected in a shift from Point A to an arterial carbon dioxide tension isobar to the left of Point A.
Changes in serum bicarbonate
Alkalemia or acidemia can also be produced by increases or decreases in the serum bicarbonate concentration, respectively. This is reflected in changes in pH with changes in bicarbonate concentration along a single carbon dioxide tension isobar (i.e., at a constant arterial carbon dioxide tension).
Changes in bicarbonate concentration or arterial carbon dioxide tension can be mitigated by concomitant changes in the other variable. An increase in serum bicarbonate has less of an effect on pH if there is a concomitant change in arterial carbon dioxide tension (increase).
Become a great clinician with our video courses and workshops
The same is true for the effect of a decrease in bicarbonate accompanied by a concomitant decrease in arterial carbon dioxide tension.
The effect on arterial pH of changes in arterial carbon dioxide tension can be mitigated by concomitant changes in serum bicarbonate, with a rise in arterial carbon dioxide tension offset by a rise in bicarbonate.
Or a decrease in arterial carbon dioxide tension offset by a decline in serum bicarbonate.
That’s it for now. If you want to improve your understanding of key concepts in medicine, and improve your clinical skills, make sure to register for a free trial account, which will give you access to free videos and downloads. We’ll help you make the right decisions for yourself and your patients.
Recommended reading
- Grippi, MA. 1995. “Gas exchange in the lung”. In: Lippincott's Pathophysiology Series: Pulmonary Pathophysiology. 1st edition. Philadelphia: Lippincott Williams & Wilkins. (Grippi 1995, 137–149)
- Grippi, MA. 1995. “Clinical presentations: gas exchange and transport”. In: Lippincott's Pathophysiology Series: Pulmonary Pathophysiology. 1st edition. Philadelphia: Lippincott Williams & Wilkins. (Grippi 1995, 171–176)
- Grippi, MA and Tino, G. 2015. “Pulmonary function testing”. In: Fishman's Pulmonary Diseases and Disorders, edited by MA, Grippi (editor-in-chief), JA, Elias, JA, Fishman, RM, Kotloff, AI, Pack, RM, Senior (editors). 5th edition. New York: McGraw-Hill Education. (Grippi and Tino 2015, 502–536)
- Tino, G and Grippi, MA. 1995. “Gas transport to and from peripheral tissues”. In: Lippincott's Pathophysiology Series: Pulmonary Pathophysiology. 1st edition. Philadelphia: Lippincott Williams & Wilkins. (Tino and Grippi 1995, 151–170)
- Wagner, PD. 2015. The physiologic basis of pulmonary gas exchange: implications for clinical interpretation of arterial blood gases. Eur Respir J. 45: 227–243. PMID: 25323225
Become an expert
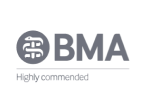
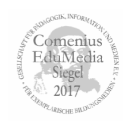